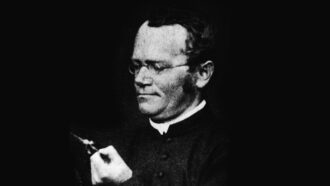
The year was 1900. Three European botanists — one Dutch, one German and one Austrian — all reported results from breeding experiments in plants. Each claimed that they had independently discovered some remarkable patterns in inheritance that had been noticed by Gregor Mendel decades earlier and reported in “Versuche über Pflanzen-Hybriden,” or “Experiments in Plant Hybridization.” All three relied on or built upon the work of the Austrian monk, whose experiments in pea plants are famous today as the foundation of genetics.
Yet at the time, “there was no such discipline as genetics, nor was there a concept of the gene,” says Yafeng Shan, a philosopher of science at the University of Kent in England. Instead, there were many theories of how traits were inherited, including Charles Darwin’s theory of pangenesis, which described particles of inheritance called “gemmules” thought to be given off by all cells in the body and to collect in the reproductive organs.
From the muddle of ideas, Shan says, those three reports at the dawn of the 20th century helped introduce Mendel’s work to other scientists in the fledgling field of heredity. That set the stage for the development of Mendelian genetics as we know it today, and no doubt played into a century’s worth of developments in molecular biology, from the discovery of the structure of DNA to the sequencing of the human genome and the rise of genetic engineering.
But the path to our current understanding of the inheritance and variation at the heart of modern biology has been far more winding than most biology textbooks reveal. In the conversation that follows, Elizabeth Quill, special projects editor at Science News, talks with Shan about the origins of genetics and what progress over the past century tells us about the nature of science.
Quill: Our understanding of genetics has emerged nearly entirely in the last century. Can you take us back? What did scientists know at the beginning of the century?
Shan: The term genetics was coined to describe the study of heredity in 1905 by the English biologist William Bateson in a letter to his friend. The term gene was introduced later, in 1909, by the Danish biologist Wilhelm Johannsen to refer to the unit of hereditary material.
That said, there were at least 30 different theories of heredity or inheritance at the beginning of the 20th century. So to borrow Charles Dickens’ phrase: It was the best of times, and it was the worst of times for the study of heredity. There were many different theories, methods and lines of inquiry available, but there was no consensus on the mechanism and patterns of inheritance, nor was there any consensus on a reliable way to study them.
Quill: In biology classes, we learn that Gregor Mendel’s experiments breeding pea plants in the mid-19th century taught us that inherited traits are delivered to offspring on pairs of genes, one from each parent, and that there are dominant and recessive forms of genes. But if the concept of gene wasn’t fully developed in Mendel’s day, what did his work actually reveal?
Shan: If you walk into any university library and pick up a copy of a genetics textbook today, you may find the following narrative: Mendel developed a theory of inheritance, but unfortunately, the theory was neglected or overlooked for over three decades, and only rediscovered in 1900.
Actually, there are mistakes in that: Mendel’s theory was not a theory of inheritance. He never used the German word for heredity — Vererbung. His concern was instead about the development of hybrids. In other words, Mendel did propose a theory for patterns of characteristics in plant hybrids, but it is not a theory of inheritance. And Mendel’s theory was not neglected or overlooked. There were more than a dozen citations to his paper before 1900. That’s not a lot, but definitely not overlooked.
Some fascinating things did happen in 1900, though. Mendel’s work was introduced to the study of heredity by Hugo de Vries, Carl Correns and Erich von Tschermak. All of them renewed Mendel’s work for different purposes. That being said, none of these three became a pioneer of Mendelism as we know it today.
Quill: Who was that pioneer?
Shan: After the introduction of Mendel’s work to the study of heredity, one important pioneer was William Bateson, an English biologist. Originally, he was not interested in the problem of heredity. So, to some extent, he was an outsider. He was studying evolution, but he found Mendel’s work useful. Based on Mendel’s findings, he said, we can develop a new theory that is the correct way to study heredity and will further shed light on the nature of evolution. He was one of the most prominent figures in the movement, which at first was resisted by many people.
To cut the story short, Mendelism won the victory — though in the early days, it was quite different from the Mendelian genetics of today, which was mainly established and developed by T.H. Morgan and his students and team at Columbia.
Quill: Thomas H. Morgan isn’t as widely known as Mendel or Darwin, for example. Why was his work so important and what made it different from what came before?
Shan: He may not have become a household name, but Morgan is considered one of the most influential geneticists ever. He actually began his career as a zoologist and had diverse interests in morphology, regeneration, embryology, et cetera. He was using fruit flies as experimental organisms to test the Darwinian theory of evolution. Darwin believed evolution happened through a series of minor and gradual changes. Others, including de Vries, believed species evolved through mutations: radical, sudden change. Morgan bought that argument.
Initially, his work was not very successful, in his own words. He started his experiment in 1908 and found nothing at all until 1910. He mentioned to an office friend that it was two years’ time, just wasted. But sometimes magical things just happen. After two years, he was surprised to find a mutation.
But he was puzzled. This mutation that he observed could not be explained by de Vries’ theory of mutation. Rather, it could be better accounted for by the Mendelian approach. So here is where Morgan and his team began developing a Mendelian approach.
What Morgan did differently from early Mendelians, say Bateson, was that he and his team incorporated Mendelism with another important line of inquiry in the field, the chromosome theory of inheritance, which was developed primarily by American geneticist Walter Sutton and German zoologist Theodor Boveri. They came up with the idea that hereditary material must be somewhere within the chromosomes. That provided a physical basis for hereditary material.
Quill: And that must have proved successful?
Shan: Combining Mendelism and the chromosome theory of inheritance leads to one of the most remarkable achievements of Morgan and his colleagues: They produced the chromosome map for the fruit fly. They located different genes at different locations on the chromosome. With that map, you can calculate the frequency of recombination of genes in the following generations. With that single map, you can identify not only the position of the genes on the chromosomes, but also predict the phenomenon of inheritance.
In 1910, Thomas Hunt Morgan (shown) and colleagues discovered a white-eyed mutant in laboratory fruit flies. Continuing work confirmed that genes, units of heredity, are located on chromosomes.
Caltech Archives, ID: 1.43-5
Quill: We haven’t yet talked about DNA. Were geneticists interested in DNA at that time?
Shan: The study of DNA was part of the job of biochemists. DNA was first identified in the mid-19th century, roughly the same time as when Mendel was working on his peas. Swiss chemist Friedrich Miescher was looking for the most fundamental constituents of life. He identified some substance coming from the nucleus of the cell and named it “nuclein.” That is what we now call DNA.
After his great discovery, the importance of and implications of nuclein, or DNA, were debated for decades. By the turn of the 20th century, nuclein was identified as a nucleic acid, and the five bases of nucleic acids — G, A, C, T and U — were also identified. In the 1920s and ’30s, biochemists came to know that the nucleic acid present in chromosomes is DNA.
But the makeup of DNA was only being pursued by biochemists. Those who studied the problem of heredity did not pay serious attention to DNA until the 1940s.
Quill: How did DNA get incorporated into the study of heredity?
Shan: That is the process of merging of the two lines of inquiry — the line of inquiry in genetics and the line of inquiry in biochemistry. For geneticists, their main concern was about a pattern and mechanism of inheritance and how a particular trait is transmitted from generation to generation. And on the other hand, biochemists were looking for the physical foundations of life.
With the success of T.H. Morgan and his colleagues, geneticists had a better capacity to predict and explain the patterns of inheritance. Then an immediate question arose: So, what are genes?
According to the Morgan school of classical genetics, a gene is just a segment of the chromosome. That’s very easy. There was very popular analogy in which they described genes as beads on the string. But it was still quite unclear what the physical basis was.
Oswald Avery and his colleagues reported evidence in 1944 that DNA, rather than protein, carries hereditary information. Even though Avery’s experiment was not actually the first — it was confirming work done by others in 1939 — his work was better received and better known within the community. People often refer to Avery’s great experiment, though at the time some skepticism remained.
Quill: That background helps explain why the discovery of the double-helix structure of DNA, from James Watson and Francis Crick, along with Rosalind Franklin and Maurice Wilkins, was so monumental. By knowing the structure of DNA, people could think about how the physical process of inheritance might work. Is that right?
Shan: Today we say, ‘Ah, so the process of inheritance is quite straightforward: Basically, DNA can be transcribed to RNA, and RNA can be translated into protein, and protein is responsible for phenotypic traits.’ Roughly speaking, it is like that.
That double-helix model provided a very reliable and useful framework to study DNA replication, and transcription. That’s crucially important for the later work in molecular genetics. At the time, in 1953, when Watson and Crick proposed that model, their work was not immediately well-received. It was not cited a lot — just like Mendel’s paper — until the end of the 1950s, when other work confirmed that the structure of DNA provides a mechanism of controlling protein synthesis.
There are quite a lot of important discoveries that followed. It’s probably unfair, but from my point of view, the others aren’t as exciting as the discovery of the double helix. If I can borrow a phrase from American philosopher Thomas Kuhn, we are now in the period of “normal science,” or what he calls “mopping up.” It took another 40 or 50 years to get where we are now, but in terms of milestones in the history of genetics, if you ask me if there’s anything as important as the introduction of Mendel’s work and the discovery of the double helix, I would say I’m afraid nothing else is as fascinating.
In 1953, James Watson (left) and Francis Crick (right) reported the discovery of the double-helix structure of DNA.A. Barrington Brown/Science Source
Quill: Looking back at the history of genetics, are there lessons to take away in how we think about science and scientific progress?
Shan: When we look back, we see that genetics developed through multiple parallel lines from the very beginning. We’ve got Darwin. We’ve got de Vries developing Darwin’s approach. We’ve got Francis Galton and his biometric approach, developed further by Karl Pearson and Raphael Weldon — which we didn’t even get to discuss. We’ve got Bateson borrowing ideas from Mendel. And there is also the important line of inquiry, the chromosome theory, independently developed primarily by Sutton and Boveri.
Across the century, we start from classical genetics, then molecular genetics and now epigenetics (which studies changes in an organism that result from how genes are turned on and off, rather than alterations to the DNA sequence). That’s three historical episodes. One popular interpretation is that these three historical episodes or paradigms can be viewed as three scientific revolutions. But these paradigms are interactive with each other, not destructive or revolutionary. For instance, molecular genetics arises from the need to better understand the physical basis of heredity in classical genetics. Even today, the methods of classical genetics are still used in some problems.
I think there are lessons here about the nature and the aim of science. Science seems to be often characterized as an enterprise in explaining or understanding the phenomena of the world. It’s right to say scientists do make efforts to explain and understand. But there is another essential feature of science, namely exploratory or investigative. From the very beginning, none of the geneticists of the past century probably had a very clear idea of what a good explanation, what a good theory, what a good experiment would look like.
Our understanding of inheritance improved with the development of investigative or exploratory research. Ultimately, some of science’s most important features cannot be simply captured by concepts like truth or knowledge or understanding.