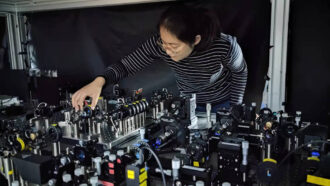
Imaginary numbers might seem like unicorns and goblins — interesting but irrelevant to reality.
But for describing matter at its roots, imaginary numbers turn out to be essential. They seem to be woven into the fabric of quantum mechanics, the math describing the realm of molecules, atoms and subatomic particles. A theory obeying the rules of quantum physics needs imaginary numbers to describe the real world, two new experiments suggest.
Imaginary numbers result from taking the square root of a negative number. They often pop up in equations as a mathematical tool to make calculations easier. But everything we can actually measure about the world is described by real numbers, the normal, nonimaginary figures we’re used to (SN: 5/8/18). That’s true in quantum physics too. Although imaginary numbers appear in the inner workings of the theory, all possible measurements generate real numbers.
Quantum theory’s prominent use of complex numbers — sums of imaginary and real numbers — was disconcerting to its founders, including physicist Erwin Schrödinger. “From the early days of quantum theory, complex numbers were treated more as a mathematical convenience than a fundamental building block,” says physicist Jingyun Fan of the Southern University of Science and Technology in Shenzhen, China.
Some physicists have attempted to build quantum theory using real numbers only, avoiding the imaginary realm with versions called “real quantum mechanics.” But without an experimental test of such theories, the question remained whether imaginary numbers were truly necessary in quantum physics, or just a useful computational tool.
A type of experiment known as a Bell test resolved a different quantum quandary, proving that quantum mechanics really requires strange quantum linkages between particles called entanglement (SN: 8/28/15). “We started thinking about whether an experiment of this sort could also refute real quantum mechanics,” says theoretical physicist Miguel Navascués of the Institute for Quantum Optics and Quantum Information Vienna. He and colleagues laid out a plan for an experiment in a paper posted online at arXiv.org in January 2021 and published December 15 in Nature.
In this plan, researchers would send pairs of entangled particles from two different sources to three different people, named according to conventional physics lingo as Alice, Bob and Charlie. Alice receives one particle, and can measure it using various settings that she chooses. Charlie does the same. Bob receives two particles and performs a special type of measurement to entangle the particles that Alice and Charlie receive. A real quantum theory, with no imaginary numbers, would predict different results than standard quantum physics, allowing the experiment to distinguish which one is correct.
Fan and colleagues performed such an experiment using photons, or particles of light, they report in a paper to be published in Physical Review Letters. By studying how Alice, Charlie and Bob’s results compare across many measurements, Fan, Navascués and colleagues show that the data could be described only by a quantum theory with complex numbers.
Another team of physicists conducted an experiment based on the same concept using a quantum computer made with superconductors, materials which conduct electricity without resistance. Those researchers, too, found that quantum physics requires complex numbers, they report in another paper to be published in Physical Review Letters. “We are curious about why complex numbers are necessary and play a fundamental role in quantum mechanics,” says quantum physicist Chao-Yang Lu of the University of Science and Technology of China in Hefei, a coauthor of the study.
But the results don’t rule out all theories that eschew imaginary numbers, notes theoretical physicist Jerry Finkelstein of Lawrence Berkeley National Laboratory in California, who was not involved with the new studies. The study eliminated certain theories based on real numbers, namely those that still follow the conventions of quantum mechanics. It’s still possible to explain the results without imaginary numbers by using a theory that breaks standard quantum rules. But those theories run into other conceptual issues, making them “ugly,” he says. But “if you’re willing to put up with the ugliness, then you can have a real quantum theory.”
Despite the caveat, other physicists agree that the quandaries raised by the new findings are compelling. “I find it intriguing when you ask questions about why is quantum mechanics the way it is,” says physicist Krister Shalm of the National Institute of Standards and Technology in Boulder, Colo. Asking whether quantum theory could be simpler or if it contains anything unnecessary, “these are very interesting and thought-provoking questions.”